Advanced nuclear: A climate-tech comeback story
Three key reasons the next generation of technology could redeem nuclear energy in the 21st century. Read More
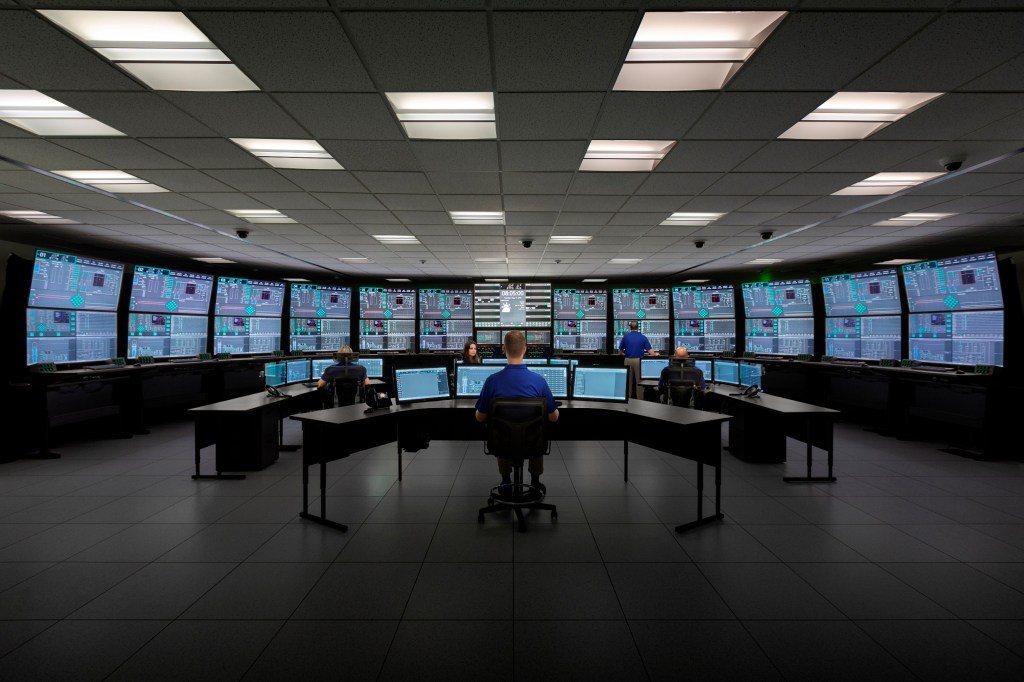
NuScale
The splitting of the atom is arguably one of humanity’s greatest technological achievements. The atom was long thought to be the smallest possible unit of matter, its name deriving from the ancient Greek for “uncuttable.” Then, in the early 20th century, scientists found a way to divide large atoms by bombarding them with wayward neutrons, releasing a colossal amount of energy. Not only can this process generate large amounts of heat and power, but it can do so without emitting climate-warming gases.
Unfortunately, almost as soon as this breakthrough was achieved, it was misused. At first, it was misused intentionally, as a means of violence. This summer marked the 75th anniversary of the United States’ bombing of Hiroshima and Nagasaki, killing 100,000 to 200,000 people in Japan. That was the only use of atomic weapons in a military conflict, but the specter of the atomic bomb has loomed large ever since, particularly during the Cold War.
Nuclear technology also has been misused unintentionally, due to negligence and poor design. Accidents such as Chernobyl, Three Mile Island and Fukushima have raised doubts about nuclear’s potential as a clean and reliable power source.
There’s no denying real and grave risks when things go wrong with nuclear power. That may be why nuclear engineering has faltered in the U.S. over the past few decades. Nuclear provides 20 percent of the U.S. electricity supply, but the country has built only one commercially operational nuclear reactor in the 21st century. Globally, the nuclear industry is expected to experience compound annual growth rates ranging between 2.5 percent (China) to 2.8 percent (Germany).
However, a new generation of nuclear technology could change the outlook. Its proponents are attempting to fix the flaws of the past and fulfill a new vision for the future, one that they hope will feature nuclear fission as a plentiful, low-carbon energy source.
“It’s a little bit like how cars or airplanes now are different from what they were 15, 20, 30, 40 years ago,” said Jacopo Buongiorno, a nuclear engineering professor at the Massachusetts Institute of Technology (MIT). “It’s the same with nuclear. You’re now buying a model 2020 Mercedes. You’re not buying a Peugeot from 1965.”
Many new models of nuclear power generation technology exist, varying in size, design and market-readiness, but they share some critical features designed to make them safer and more versatile than previous models.
Some experts are skeptical that these new technologies will be able to achieve their intended advantages at a reasonable cost, in a manner safe enough to confidently allay concerns — at least not without huge government subsidies, which seem unlikely in the U.S. for the foreseeable future.
“If it sounds too good to be true, it probably is,” said Edwin Lyman, director of nuclear power safety at the Union of Concerned Scientists.
Like any comeback story, there are major barriers to overcome, and there’s no guarantee of success. However, there’s also something captivating about a redemption arc. In particular, there are three opportunities that nuclear advocates see as a pathway to redeeming nuclear energy in the 21st century.

NuScale is making plans to build the first small-scale nuclear plant in the U.S., with 12 reactors. Photo (aerial view) courtesy of NuScale
Opportunity 1: Downsizing
One of the biggest recent advances in nuclear energy has been making reactors smaller. The reactors of the 20th century were large, expensive behemoths. These large plants could generate enough energy to power millions of homes, but they also required decades of time and billions of dollars to build.
The latest trend in nuclear is the small modular reactor (SMR), which generates a fraction of the energy of traditional reactors, but at a fraction of the cost. Existing nuclear reactors generate 500 megawatts to 1 gigawatt of electricity. SMRs generate less than 300 MW.
A benefit of smaller reactors is that they’re more flexible than their predecessors. A single reactor could suit projects with lower energy needs, and multiple reactors can be added onto projects with higher energy needs, such as hitching additional cars to a freight train or connecting more bulbs to a string of holiday lights.
NuScale, based in Oregon, arguably has made the most progress with SMR technology. Its reactors have a capacity of 60 MW, enough to power about 50,000 homes. The company recently received preliminary design approval from the U.S. Nuclear Regulatory Commission (NRC), making its design the closest to getting a green light of any small-scale nuclear reactor.
“That’s a huge milestone for us,” said Jose Reyes, chief technology officer at NuScale.
With help from a $1.35 billion grant from the Department of Energy, NuScale is making plans to build the United States’ first small-scale nuclear power project. The plant would consist of 12 reactors selling electricity to a consortium of cities in Utah, with the first reactor turning on by 2029.
“We believe we’re paving the way in terms of what we’re doing with safety and in terms of moving to this different paradigm for deployment of reactors,” Reyes said.
The Utah project still has a long way to go, and it’s been bogged down over the past few months by delayed timelines, withdrawn city partnerships and lingering questions about safety.
Although the road to advanced nuclear power is still being paved, it’s far from empty. A whole slate of companies are attempting to build next-generation nuclear power plants at the end of this decade and the beginning of the next one.
Like NuScale, many of these companies are thinking small, but some are also thinking different. NuScale’s reactor is a smaller version of the reactors at 20th-century nuclear power plants, which use water to cool the high-temperature reactions at the core. Newer technologies cool the reactions with molten salt or high-temperature gas.
Terrestrial Energy, based in Ontario, is working with Canadian regulatory agencies to get approval for its molten salt reactor design. The company aims to have two plants licensed and built by 2030, each with a capacity of 195 MW.
Canon Bryan, chief financial officer of Terrestrial, noted that building small isn’t just a practical opportunity, it’s a financial one. He has observed that private investors are hesitant to back a project that would cost over $1 billion, but expenditures less than that are a different story.
“The single biggest constraint that faces the nuclear energy industry globally today, in terms of deployment, is upfront cost,” he said.
Buongiorno also sees cost as a critical threshold, especially when small nuclear plants are feeding into the electric grid, competing with other sources of electricity generation but also complementing them.
“They would be a good combo with renewables,” he said, “because when the sun doesn’t shine, the wind doesn’t blow, you can have your nuclear reactors that cover the peaks and the valleys. The key question is, at what cost? If the levelized cost of electricity for this system is too high then people will just not buy them, and they might find it even more advantageous to buy big storage.”
For off-grid applications, some nuclear technology companies are thinking even smaller than small. A few companies are working on “micro”-scale reactors of less than 50 MW, with some as small as 1 MW. These microreactors could power mines as well as unconnected or poorly connected communities.
One such company is Ultra Safe Nuclear Corporation (USNC), which has developed a helium-cooled microreactor with 5 MW capacity. Mark Mitchell, president of USNC’s power division, noted that starting tiny and getting bigger is the same pathway that worked for renewable energy such as solar.
“It represents a new way of doing nuclear strongly modeled on how renewables businesses have worked and scaled over the last 10 years,” he said. “If nuclear learns the lessons from renewables, I think it will be very powerful.”
Bryan emphasized that having a variety of sizes opens the door to a wide set of applications for nuclear, in a way that was previously impossible. “The only types of nuclear power plants that are available today are one size: enormous, and one size does not fit all,” he said
Opportunity 2: Versatility
When it comes to energy generation, electricity is just the beginning. More than 80 percent of the world’s energy goes towards non-electricity uses such as industrial processes and transportation. In that sense, the nuclear plants of the 20th century essentially have been humongous one-trick ponies, because their only function is to make electricity. The next generation of nuclear has the potential to do other tricks, and the basis for most of those tricks is heat.
“Most everything we encounter in society is made in an industrial facility, and most every single industrial facility uses heat,” Bryan said. “That is a vast market that nuclear innovation can compete in, where no other technology really can, except for fossil fuels. If nuclear innovation can compete on cost with fossil fuels, then I would have to say that it’s game, set, match.”
Traditional reactors can’t exceed 572 degrees Fahrenheit or the coolant will start to boil off too quickly, creating major safety risks. However, that’s not a problem for molten salt reactors, which can reach temperatures of 1,292 degrees F, or high-temperature gas reactors, which can reach over 1,652 degrees F.
Bryan said the large majority of industrial facilities require 25 to 150 MW of energy, which means that in most cases, small reactors are at least theoretically capable of replacing fossil fuels to meet industrial demand, as long as they can get costs down.
And that’s just the industrial processes of today. A low-carbon future will require a whole new set of energy-intensive processes. Combining heat, steam and electricity is a potent recipe for pulling out the hydrogen from water and using it for hydrogen fuel. Heat is critical to manufacturing steel and concrete, some of the most challenging areas to decarbonize. In order to have a net benefit, capturing CO2 directly from the atmosphere requires low-carbon sources of either heat or electricity. All of these processes potentially could be powered by small, high-temperature nuclear reactors.
“If you can use [nuclear reactors], then you’re going to be able to start to decarbonize sectors that otherwise, just with electricity, you wouldn’t be able to touch,” Buongiorno said.
However, safety experts such as Lyman warn that industrial applications bring additional risks. Heat-producing reactors would need to be in close proximity to the facilities where the heat is used, making proper containment procedures even more critical.
“If you’re going to be locating nuclear reactors close to chemical facilities that are flammable or combustible, obviously that can infuse new concerns,” Lyman said.

Canadian firm Terrestrial Energy is designing small-scale molten salt reactors. It hopes to have two plants, each with a 195-megawatt capacity, licensed and built by 2030. Courtesy of Terrestrial Energy
Opportunity 3: Safer by design
From a climate perspective, it’s encouraging to imagine a future filled with small nuclear plants furnishing cheap, plentiful, low-carbon energy for a wide variety of purposes. However, some will find it unsettling to live near a small nuclear plant. Nuclear technologies need to assure safety beyond any shadow of a doubt. That’s why advanced nuclear companies are planning inherent safety features that they hope will make many potential calamities not just improbable but impossible.
The safety risks can be grouped generally into three main categories. First, there’s the risk that nuclear fuel will fall into the wrong hands and be used to create a nuclear weapon. Then, there’s the risk that a plant will malfunction and release toxic radiation. Lastly, there’s the risk that the leftover material will pose a danger wherever it’s stored.
Let’s start with measures to address the risk of weapons proliferation. Any radioactive substance needs to be vigilantly handled, but the fuel used in most nuclear reactors is very different from weapons-grade nuclear material. The concentration of radioactive uranium is far lower, and the enrichment process to get a higher concentration is complicated and expensive, not something that could be done by any individual actor.
Furthermore, a benefit of smaller reactors is that they’re transportable. That means that even if the reactors themselves are widely distributed, they can be designed such that the fuel is only added or removed in a central, secure location.
These elements don’t rule out the risk of nuclear material getting into the wrong hands, but they help ensure a nuclear power sector that’s distinct from weapons production.
Next, there’s the risk of disastrous accidents. Each failure that’s happened in the past has been a learning experience, leading to enhanced designs that aim to remove the possibility of the same problems reoccurring, even in extreme or unlikely conditions. This is called inherent safety.
For instance, the Fukushima meltdown happened after a tsunami knocked out the electricity supply. Advanced nuclear designs use passive cooling techniques that rely on gravity and don’t require external power. In Fukushima, the water used to cool the plant boiled off. Small nuclear reactors such as NuScale’s are designed to cool from the surrounding air if there’s no water around them, and as mentioned previously, coolants such as molten salt and high-temperature gas don’t boil off.
Ultimately, what happens when reactors fail is that the solid fuel melts and seeps out of the area where it’s contained, hence the term “meltdown.” Gas reactors use fuel balls that are each individually coated with a protective shield, and most molten salt reactors don’t use solid fuel at all. Instead, their fuel is dissolved in the salt, which removes the meltdown risk entirely.
“You can’t melt down something that is already liquid,” Bryan said.
However, these new coolants have their own challenges and complications, such as safeguards for containing high-temperature processes or accounting for byproducts. Traditional reactors have developed multiple layers of protection, and advanced technologies likely would need to sort out additional safety mechanisms to complement what’s already built into the design.
“If you give too much credit to fundamental design features for safety and then use that to justify reducing other types of safety measures, then you end up with something that’s less safe,” Lyman said.
Lastly, there’s the waste, which nuclear professionals refer to as “spent fuel.” Some reactors use what’s called a fast reaction, which converts spent fuel back into usable material, leading to an end product that’s much less radioactive, or potentially to no extra material whatsoever, if this process is repeated a sufficient number of times.
However, fast reactors are harder to stabilize than other reactors, because other reactors have built-in mechanisms to slow and control the reaction. Fast reactors also can operate with weapons-grade plutonium fuel, which can be a useful way to permanently get rid of weapons stocks but also creates a risk of additional proliferation.
In practice, most advanced reactors likely would create some sort of spent fuel that would need to be stored securely for centuries. Currently, in the U.S., spent fuel is stored onsite at nuclear plants, which isn’t sustainable in the long term.
Finding a more permanent place to store this spent fuel has been tricky. Most notably, the plans for a repository at Yucca Mountain in Nevada have been delayed indefinitely due to local opposition.
“The issue has always been the political process and in the U.S., we’ve done it disastrously wrong,” Buongiorno said.
However, European countries such as Finland are already building storage sites, which have moved forward with the support and buy-in of the surrounding communities. Once repositories such as these are built, they only need to store a modest volume of spent fuel.
Buongiorno has a knack for coming up with clever visualizations, and he helped me to put the volume of spent fuel into context. “If you or I were to use nuclear energy for our lifetime, the amount of high-level waste … would fit into a teacup,” he said. “All your life, just nuclear electricity, that’s the amount of waste that you have.”
Obviously, those teacups will add up, and they’re radioactive, but if the politics can be sorted out, it’s a manageable amount of material, which is true for both advanced reactors and traditional ones.
Still, it’s not everyone’s cup of tea. All of these safety measures can’t guarantee that advanced nuclear will be absolutely risk-free, especially as there aren’t yet any advanced nuclear plants in commercial operation. There’s always the potential for unknown unknowns with new technology, and in the case of nuclear energy, mistakes can be catastrophic. The fact that older technologies already have experienced errors and shutdowns doesn’t help.
“One of the key considerations when you talk about developing an entire new class of reactor that doesn’t have any operating experience is trying to take into account all the uncertainties,” Lyman said.
Every nuclear technology company I spoke with emphasized taking nuclear safety seriously. Ultra Safe Nuclear Corporation (USNC) even chose to put safety front and center by including it as part of its name.
“Our name is a challenge to ourselves and the industry as well,” Mitchell said.
This safety-first approach goes beyond lip service. Companies need to show thorough evidence of safety in order to receive the regulatory approval necessary for setting up a new nuclear plant in countries such as the U.S. and Canada.
Lyman, however, is worried that nuclear companies and the NRC are rushing the process to bring unproven technologies to market.
“The NRC is under quite a bit of pressure by the industry and by Congress not to hold things up,” he said.
In addition to the formal regulatory process, there’s also the court of public opinion, an additional reason for companies to think through every possible risk, even the ones that seem improbable.
“They’re perceived issues,” said Carl Perez, chief executive officer of Elysium Industries, a company that has designed a large-scale molten salt reactor. “And that’s enough to consider it a problem. At the end of the day, social licenses are really at the forefront of reactor design.”

Rendering of the Oklo microreactor. Courtesy of Oklo
Nuclear’s next act
Nuclear power has taken a backstage role to renewables as a clean and promising energy option, but it’s on the verge of entering back into the limelight, potentially much sooner than some might expect.
“A lot of the general thinking out there is that these technologies are great, but they’re not going to be ready for 30, 40, 50 years. What we’re here to say is that that is rubbish,” Bryan said.
Even nuclear fusion, the high-energy combination of small atoms (such as what happens inside the sun), is seeming more within the realm of possibility.
This technological progress is exciting because nuclear energy is seen by some as an important tool to have in the toolbelt for solving climate change. Buongiorno and a team of other nuclear researchers ran scenarios for reducing emissions in the electricity sector in multiple regions of the world, and they found that advanced nuclear fission was critical to reaching the lowest carbon at the lowest cost.
“Nuclear really can and should play a role if we’re serious about decarbonizing,” Buongiorno said.
The Union of Concerned Scientists, which has long been critical of nuclear technologies, also recognizes the value of nuclear power as a carbon-free energy source, although the organization continues to push for the strictest possible safety considerations.
“If you start off by saying you have to have nuclear power as part of the system no matter what, then you could come up with the wrong answer with regard to the level of safety you need,” Lyman said.
Some nuclear advocates see decarbonization as an imperative to think bigger. Perez is skeptical of small modular reactors. His company is looking to build large molten salt reactors (more than 1 GW) in parts of the world where energy demand is increasing rapidly.
“How quickly do we want to decarbonize the world? Because if we want to do so quickly, then we need to not build small output reactors,” he said.
However, decarbonization is a motivating factor for developing smaller technologies as well. Oklo has designed a 1.5 MW microreactor, and its founders also see the company’s technology as a part of the patchwork of solutions necessary to address climate change.
“Countries that have deeply decarbonized did it using nuclear and renewables,” said Caroline Cochran, chief operating officer of Oklo. “That’s what we really need to do if we want to rapidly decarbonize in a way that we have to do if we want any semblance of not having massive ecosystem collapse.”
Oklo takes its name from the Oklo region of Gabon, where uranium deposits have formed natural fission reactors for 2 billion years, deep below the earth’s surface. This name choice is a reminder that nuclear fission has been a part of the world for eons, even though humanity only stumbled onto it recently.
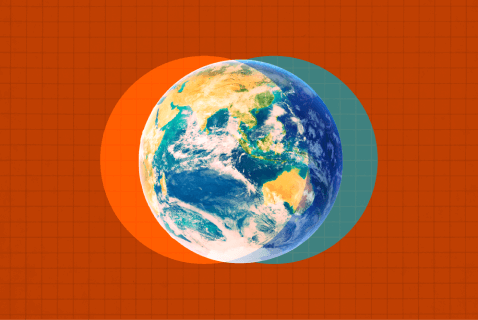